Geological Materials
Geo-materials are those that are natural occurring, heterogeneous, contain flaws at varying size scales and are weak in tension Their behavior is difficult to predict under varying strain rates and stress conditions because of their heterogeneity, multiphase character, and inherent flaws, fissures, fractures, and joints. Cements and concretes also exhibit similar characteristics. In some instances, geo-materials can flow and behave like fluids under high strain rates or conditions that cause the loss of shear strength (liquefaction). In other instances represent brittle behavior. Because of these properties and flaws, geo-materials differ fundamentally from metals, polymers and other materials. These reasons have motivated our center to concentrate on advancing the state of the science (or art) in observing, modeling, simulating, designing and predicting the complex behavior of geo-materials. Our center will be a focus of expertise in observational and experimental science to develop both theoretical understanding and numerical tools for analysis, simulation and design to address several pressing national issues. These issues involve the nation's energy independence, impact to environment., and development of new materials for safety and endurance.
Energy Independence
The US is experiencing a boom in the development of unconventional fossil energy and alternative energy. From unconventional natural gas and oil development perspective, there are issues that are directly being addressed by our center from observation of micro-seismic emission during hydraulic fracturing to extensive experimental measurements to characterize geo-materials (e.g. shale, sandstone and carbonates) to detailed numerical simulation of the response of geo-materials. Our center brings experience in theoretical and numerical implementation of state of the art numerical models for advancing our understanding of how rock and engineered geo-materials fail under different thermal, hydraulic, pressure and chemical (THMC) regimes. Enhanced geothermal systems (dry) and conventional hydrothermal energy reservoirs are continually monitored for seismic emissions and in some cases have been shut down due to uncertainties in the hazard associated with induced seismicity. Geothermal systems must be engineered to be safe and reliable which can only be achieved if there is a thorough understanding of the behavior of geo-materials (e.g. granite, shale and cementitious materials) in extreme environments. The center has at its core expertise in fracturing of natural and man-made materials including degradation due to THMC processes over the life of a natural geothermal reservoir.
Environmental Issues
Associated with some of the above energy issues come adverse impacts to the natural environment. Waste water produced as a by-product of unconventional oils and gas production must be either cleaned or disposed of by deep injection. The most common process is deep injection. However, this process may be associated with undesirable induced seismicity. Understanding how deep injection can create induced seismicity and associated risk is an important issue and is one focus of our center.
Why focus first on Unconventional Oil and Gas
Tight shale gas is an unconventional fossil energy that is already affecting US energy independence with reserves projected to last for many decades to come. The availability of shale gas in many diverse locations throughout the United States and its use to replace coal power plants has led to lower levels of CO2 emissions. Production of methane and other hydrocarbons from low permeability shale involves hydraulic fracturing of rock, establishing fracture connectivity, and multiphase fluid-flow and reaction processes, all of which are currently poorly understood. Fracturing phenomena involve fluid-solid interactions embedded within coupled thermo-hydro-mechanical-chemical processes over scales from microns to tens of meters. Crack propagation in dry materials has been studied, yet the role of fluids and its chemistry in propagating fractures is not well understood.
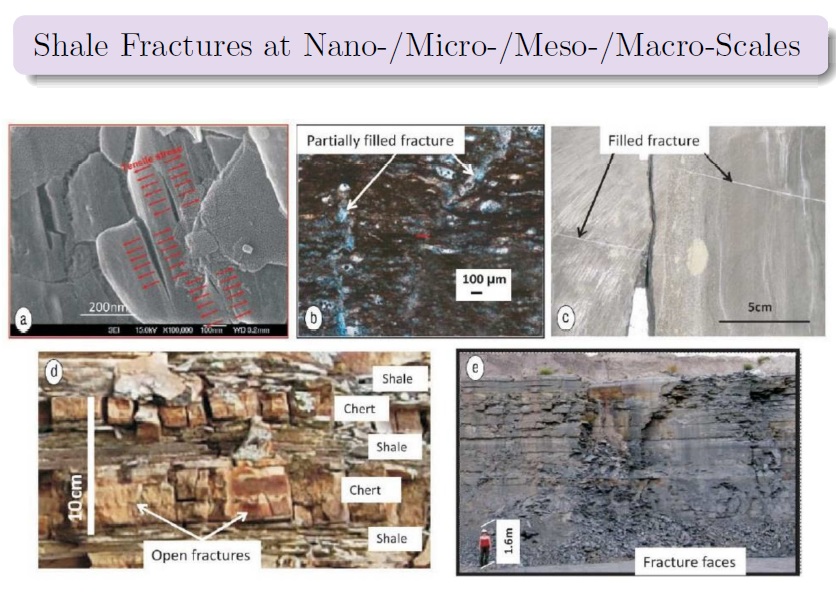
Shale, the sealing formations in most hydrocarbon reservoirs, is made of highly compacted clay particles of sub-micrometer size, nanometric porosity and various mineralogy. It is probably one of the most complicated and intriguing natural material present on earth. The multiphase composition is permanently evolving over various scales of length and time, creating in the course of this process the most heterogeneous class of materials in existence. The heterogeneities manifest themselves from the nanoscale to the macroscopic scale, which all contribute to a pronounced anisotropy and large variety of macroscopic behavior. Images from the Leading Edge, 30, 274 (2011).
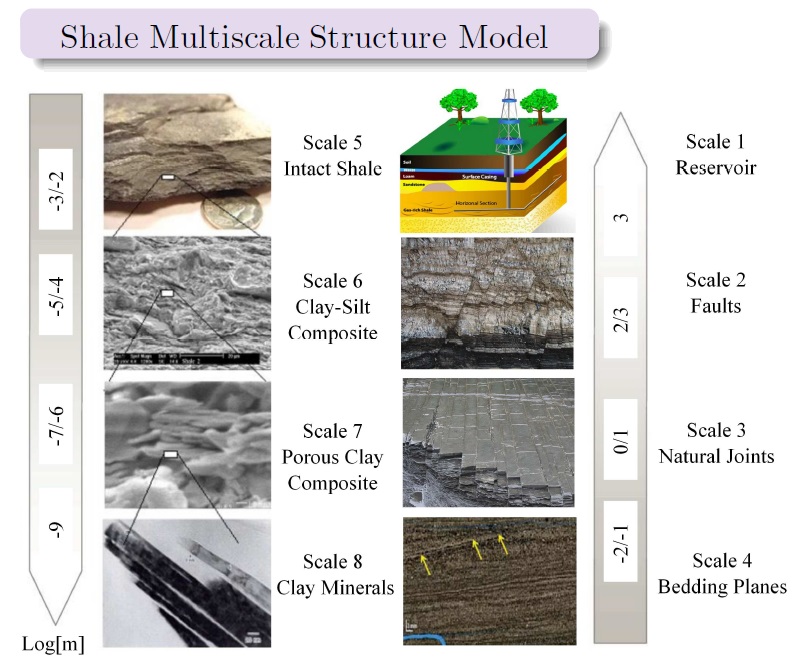
The multiscale structure model provides a consistent framework for separating the length scales that are most relevant to our research. The formulation of a multiscale simulation model requires three fundamental ingredients: (1) mathematical formulation describing the behavior of interest at a certain given scale; (2) experimental and field observations for the purpose of calibration and validation; and (3) multiscale theories and technologies allowing the upscaling of the selected model to Scale 1. Since the goal of our research is to obtain theories and models to be used at Scale 1 for practical applications, we establish the length scales of interest to be that of an intact rock specimen at the centimeter scale and higher, i.e., from Scale 4 to Scale 1. Other finer scales defined all the way down to the atomic structure are not scales of our interest. Images for Scales 5-8 are from Geophys. Res. Lett. 74, D65 (2009).
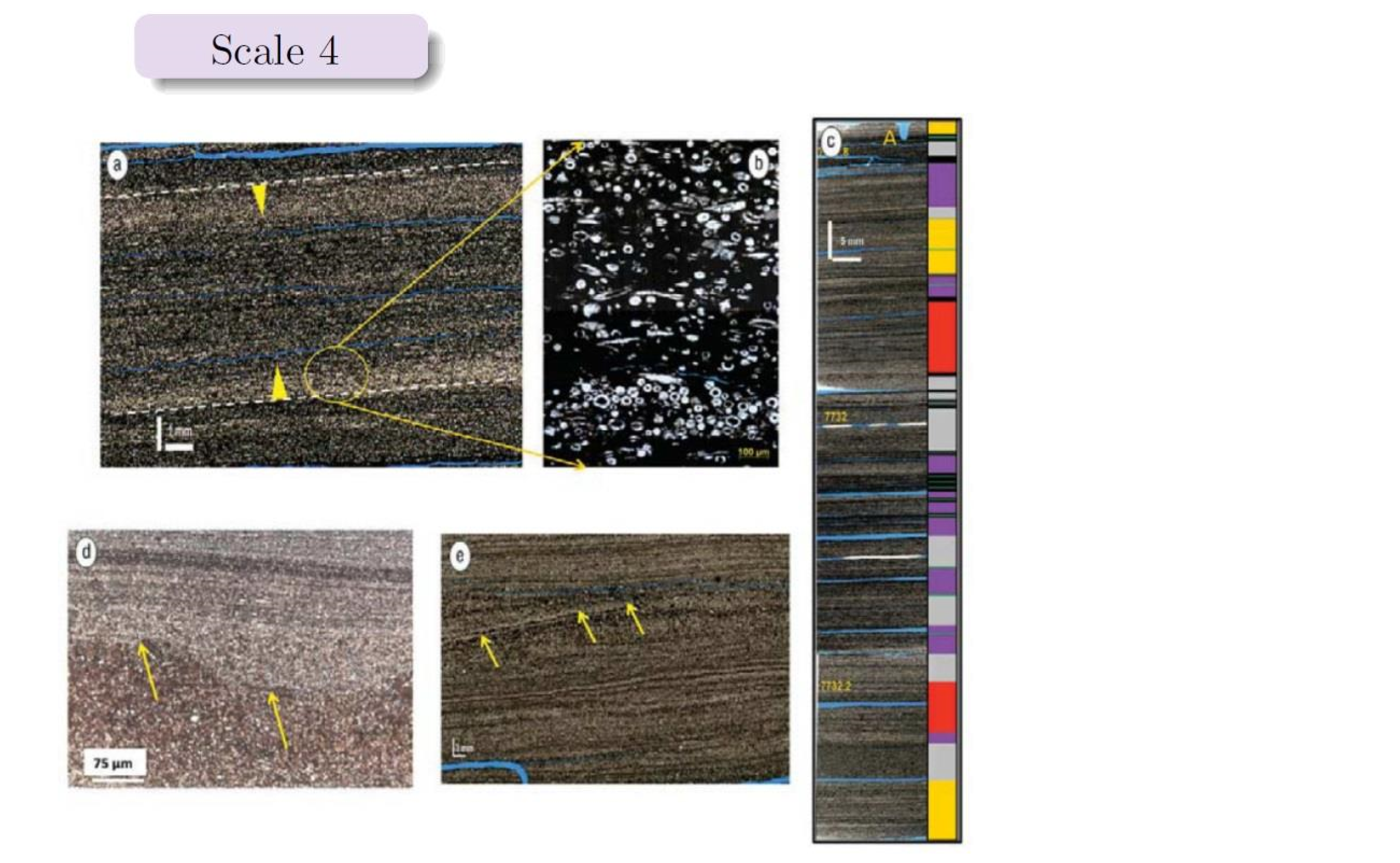
Scale 4 corresponds to that of a typical laboratory specimen used in standard mechanical characterization experiments such as acoustic measurements for elasticity and triaxial testing for strength properties. As a result of sediment transport by a variety of processes, many shale specimens exhibit mm- and cm-scale grain-size variability, lamination, bedding planes, scour surfaces, and burrows, which play a significant role in shale geomechanical characteristics, such as the degree of anisotropy, tensile strength and fracture toughness. The light objects, about 100 microns in typical size, are silt inclusions randomly distributed throughout the composite. Images from the Leading Edge, 30, 274 (2011).
At Scale 3 and Scale 2, the mechanical and hydraulic properties of many rock masses are significantly affected by the additional mechanical compliance and fluid conductivity that result from joints and faults. Depending on structure, porosity and geometry, faults may act either as seals or preferential pathways for fluid flow. For example, faults can affect hydraulic stimulations channelized to propagate along faults. A fault can effectively dominate the fracture growth and redirect all the energy of the treatment into the fault system and out of the target zone. The injection-induced seismicity can occasionally travel upwards thousands of feet, in most cases caused by inducing fracturing along the faults. Images from Marcellus Shale and GoogleEarth.
Because of shale’s unique and very intricate geological, physical and mechanical properties compared with sandstones and carbonates, the adjective “unconventional” has often been applied to their characterization. Despite the remarkable interest in their properties, the current literature on reservoir modeling exhibits considerable gaps. The research community is still hampered by the lack of capabilities in dealing with inherent and emergent heterogeneities, as well as to quantify the effects of the microstructure on failure events. The typical tools of petroleum engineering ignore the heterogeneous responses, and stretch traditional continuum mechanics beyond its limits of applicability. Conventional theories such as elasticity and plasticity lose their usual meaning under strongly heterogeneous conditions and must be reconsidered in light of the evolving microstructure. At present, there are no convincing mechanical methodologies able to predict mesocale phenomena, capture the evolution of heterogeneities, and use this information to derive microstructural continuum models for reservoir engineering. Simultaneous use of continuum and discrete methods is rarely pursued. The key goal of our work is to derive a new generation of numerical simulators for hydraulic fracturing able to link its macroscopic fracture network with the microscopic scale at which they actually take place. In our approach, continuum and discrete models are regarded as complementary, and therefore mutually interfaced to achieve reliable yet efficient reservoir modeling.